Glaucia Mendes Souzaa†, Luciano M. Verdadea, Carlos Henrique de Brito Cruzb, Stephen Kaffkac, Patricia Osseweijerd, Chris R. Somervillee, and Heather Youngse
Contact: †glmsouza@iq.usp.br
aUniversidade de São Paulo, Brazil;
bSão Paulo Research Foundation (FAPESP), and University of Campinas, Brazil;
cUniversity of California Davis, USA;
dDelft University of Technology, The Netherlands;
eUniversity of California Berkeley, USA
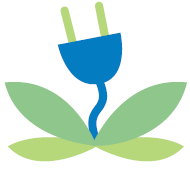
SCOPE Bioenergy & Sustainability is a collective effort with contributions from 137 researchers from 82 institutions in 24 countries. The volume is the outcome of a Rapid Assessment Process that included a meeting at UNESCO, Paris, in December 2013 where 50 experts discussed bioenergy sustainability crosscutting aspects. For additional details refer to the synthesis volume that includes background commissioned chapters to provide context,issues, latest developments and the much-needed science regarding bioenergy expansion.
Integration of Sciences for Bioenergy to Achieve its Maximum Benefits
SCOPE Bioenergy & Sustainability reports on recent technological advances, scientific developments and challenges for bioenergy expansion across its whole lifeline considering land use, feedstocks, conversion technologies and impacts. It covers all products of bioenergy production and describes added benefits to energy security, food security, environmental and climate security, sustainable development and innovation. It also aims to define the next research steps, the much-needed science where gaps of knowledge exist.
There are several challenges that need to be addressed in bioenergy so that it achieves its maximum benefits. They should preferably be assessed in an integrated manner. Factors that limit the positive impact of bioenergy to global energy security are (1) the availability of sustainable biomass, (2) non-polluting, energy positive, and cost-effective conversion technologies, and (3) the societal aspects1. Factors supporting increased utilization of bioenergy as a pathway towards improved food security should also be considered, since bioenergy is only one of many aspects that can affect food security. Good methods for monitoring food security need to be developed. Methods for identifying win-win situations as well as trade-offs for integrated Food-Energy systems are required2. Conversion technologies that use biomass carbons with great efficiency such as in the production of ethanol from starch and sugar or fatty acid methyl ester (FAME) biodiesel exist today, but for other applications many pathways are still developmental or too expensive3.
In addition to research and data collection, assessment frameworks are required to support the integration of bioenergy systems into existing agriculture and forestry practices. Such strategy needs broad stakeholder involvement in order to capture synergies and strike a balance between social, economic and environmental objectives.
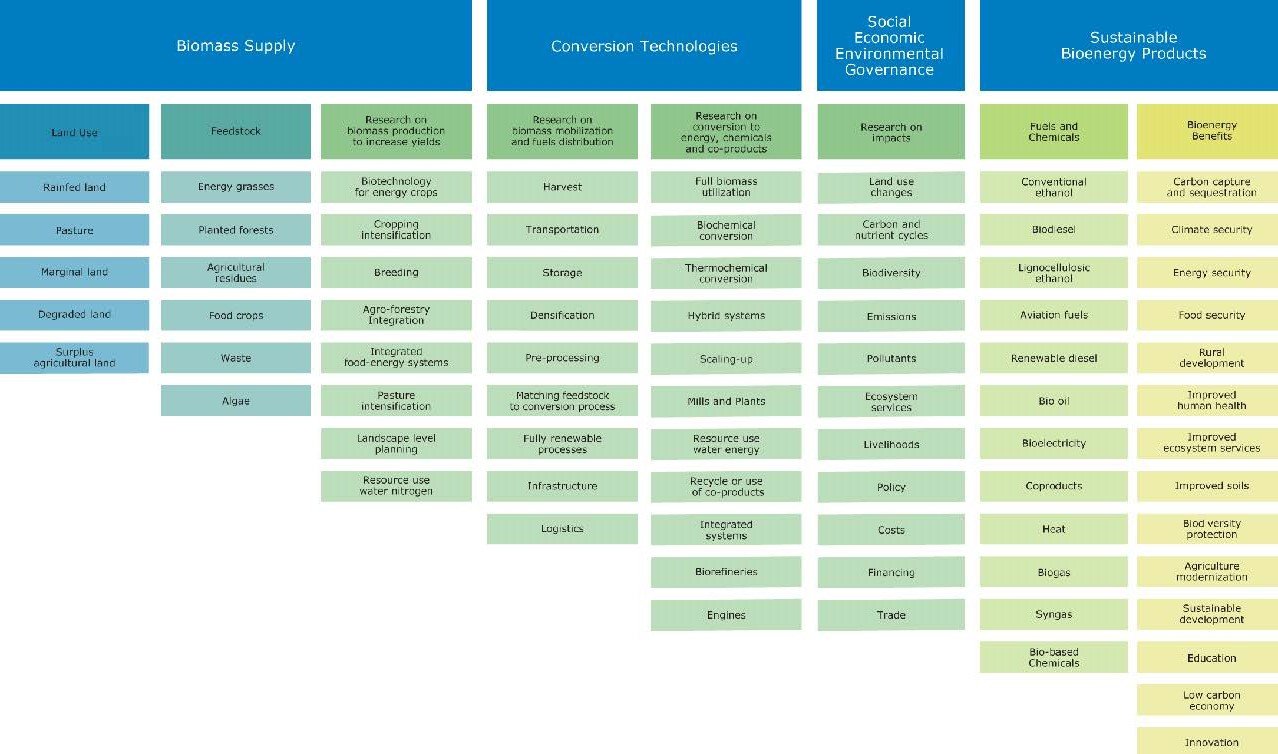
The development and use of relevant indicators is one critical part of this work4.
There is need for social science research into the preconditions, processes, and governance required so that emerging bioenergy models grow and thrive. Dissemination tools need to be developed. Challenges are often not technical, but relate to educational resources, social and cultural norms, private and public financing, infrastructure, markets, policy and governance5. Governance has been identified as a key factor for bioenergy to achieve its positive effects on food security and climate change6.
In addition to governance we also require insight in financing models for improved, more sustainable agricultural systems. New finance and investment models are
needed. Data on best practices should increase our insight on improved schemes for financing as well as on the way this should be governed or organized7. Economic models should be enhanced to better quantify the indirect land use and rebound effects of the bioeconomy, understand better the impact of bioenergy on the various dimensions of food security, and to improve the modeling of technological change8.
Communication and mutual learning will be very important in the transition to a more sustainable global energy matrix. Novel ways of communication, especially on how to involve the public, need to be designed. Communication of factual data on how bioenergy can improve food security to public(s) in general should be designed in such a way that it takes the negative and wrong assumptions away and decreases the negative impact of public opinion on policy and decision makers. This requires input from communication sciences and ethics. At a higher level, we need knowledge on institutional arrangements, the interplay between local, regional, national and global schemes, international relations, market and management studies, with understanding of impacts in agriculture for bioenergy, feed and food production9. We need to build capacity directed at the diverse networks that support biomass, bioenergy, and biofuels implementation to facilitate governance, research, public understanding of the different options and their impacts, and improve awareness of environment, safety and health implications across supply chains. Bioenergy production schemes could profit from international cooperation to adequately be deployed, while still accounting for diverse economic and agricultural circumstances of different countries, including infrastructure for current local fuel distribution and use, vehicle and jet plane manufacturing facilities and use, cultural practices, regulations and their enforcement, and a number of other factors.
7.1 Policy
Evidence is needed on the effectiveness of policies with regard to the various sustainability dimensions (people, profit, planet and governance)10. Studies should also address what policy measures are needed at local and global scales and which ones are most likely to be effective in the long-term. Research is needed to understand the influence of regional sociopolitical landscapes, technology readiness, economic resilience, infrastructure capacity, and maturation of environmental awareness among stakeholders on both the scope and timing of effective policies. With increasing globalization, research must be undertaken to bring knowledge on the implications of global bioenergy trade including the implications of multilateral agreements on energy and climate11. Holding emerging bioenergy to higher standards than the current agriculture and forestry ones may inhibit, not aid, emerging and more sustainable opportunities12 and studies on policy are needed to define a comprehensive beneficial policy framework. Knowledge and theory are needed to craft policies that enable transitions to sustainability within a context of minimizing uncertainty and maximizing positive social outcomes for current and future generations.
7.2 Sustainable Biomass Supply
Understanding the potential uses of degraded land, pasture lands, lands that were previously used for food and/or cash crop production and are currently abandoned land, and those that are only marginally suitable or unsuitable for food and/or cash crop production requires new comprehensive, integrated, and where possible, landscape-level research approaches13. It is not currently possible to clearly distinguish among these categories of land14. Understanding intersections between biophysical, agronomic, and economic constraints, along with environmental impacts of change in the various possible uses of these lands, are key knowledge gaps.
Increased trials of bioenergy crops in environments where bioenergy expansion is anticipated are needed to provide data on crop performance in target environments before their wider expansion15.
The decision making process could benefit from modeling approaches in the identification of optimal locations for energy crops16. The availability of yield models and empirical verification could help define available areas for bioenergy crops cultivation, including subsequent efficient crop handling17. These will require the development of applied research and extension capacity. A robust research and extension system focused on constant improvement in farming practices, including the impacts of different scales of operation, is an essential feature of an agriculturally based bioenergy economy. Research on effective land management with a focus on yields and sustainable practices should inform agriculture worldwide and include the development of markets for agricultural products18. Such models should also take into account regional social benefits; this requires insight in social benefit accumulation of introductions of bioenergy production systems.
Studies will have to identify improved yields, in parallel with better water and nutrient management while generating insight on the required scale of operations19. Long-term studies on use of ash and biochar as fertilizers and soil amendments should be supported20.
We need a robust research and extension system focused on constant improvement in farming practices, including the impacts of different scales of operation. Research on effective management of land and water with a focus on yields and sustainable practices should inform agriculture worldwide and include the development of markets for agricultural products21.
We need integrated assessments of social, environmental, economic aspects of bioenergy systems for a sustainable supply of biomass, adopting a landscape approach
of natural resources management22. This approach requires multidisciplinary studies in which agronomy, economics, management, bioprocess engineering and social studies come together to provide input to fully understand the value chains in specific regions23.
The mechanisms and magnitude of the productivity of diverse, agro-forestry integrated systems relative to uniform monocultures remain contested and need further studies. Fundamental research on ecological principles, possibilities of cropping intensification, along with systems research on specific mixtures and integration strategies in different socio-ecological contexts, is needed to quantify the costs, advantages, and trade-offs of integration. These studies should be done with a common set of metrics, so that valid comparison to other studies in other regions is possible, and eventually so that a meta-analysis of the results can be made24. Impacts of agroforestry residues on the soil resources, pest populations and disease dynamics must be better understood25.
7.3 Feedstocks
Climate change can alter biomass production for some crops and hinder recent yield gains. Breeding for resource-use efficiency (water-use and nitrogen-use efficiency) and “future climate-resilient” bioenergy crops should be stimulated, including tolerance to drought, temperature extremes, water-logging and salt accumulation26.
Using biotechnology, maize production in the USA has achieved impressive yield gains but in other parts of the world, maize yields are low. Sugarcane and perennial energy crops are far from theoretical yield potentials. Using either marker-assisted breeding and conventional approaches or the GM route, energy crops biotechnological development is desired27. Long-term studies of perennial bioenergy crops and shortrotation forests in relation to ecosystem services, including biodiversity, water quality and availability, and soil carbon are needed28. More extensive trials with a range of agronomies for emerging perennial crops to test the assumption that these will be high yielding and sustainable on marginal land and other areas unsuitable to competitive food crop production are also needed29.
The appropriate fraction of biomass residues that should be left in fields for preserving the agronomic benefits should be investigated for specific crops to provide opportunities for collecting part of it for bioenergy production. The nutrients obtained in the form of ash or sludge in processing plants should be investigated for their potential reuse or recycling to fields as good sustainable practices30.
There is also need for research into barriers to adoption of new and emerging crops by farmers31.
7.4 Logistics
The internal or captive use of renewable energy in biorefineries should be investigated and promoted in biomass supply chains to make the overall biomass to bioenergy system sustainable32.
Alternative modes of biomass harvesting, transportation and storage should also be developed to improve soil quality, energy balances and adequacy of the
biomass delivered to processing facilities. This should include evaluation of factoryscale in relation to biomass transport. Supportive technology and mechanization improvements are needed. Appropriate biomass densification techniques and equipment that can handle multiple types of biomass should be developed and deployed for field operations to improve transportation and storage for upfront processing. Other techniques such as pelletisation should be deployed while
emerging ones like torrefaction should be investigated with respect to its technical performance and economics. Low cost in-field biomass drying options should be investigated in an attempt to reduce any equipment and energy-intensive upfront processing in bioenergy processing plants; on the other hand moisture tolerant
conversion technologies should be developed33.
In developed countries studies are needed as to how best synch up the bioenergy forms under development with the existing infrastructure. Studies are needed as to which sustainable infrastructure can be deployed to improve the availability of biomass in developing countries34. In all regions, research to understand how electricity from biomass can integrate in the future renewable energy supply is needed. Electricity from biomass can serve in either a baseload or firming capacity (through biogas), thus providing crucial support to intermittent sources of electricity such as wind and solar, which can allow greater system-wide efficiencies. Understanding trade-offs in distributed versus centralized electricity and the role of biomass is also an emerging gap. Research in system-wide leveraging of heat35 and electricity at bioprocessing centers for additional industrial activities or community level energy access and security should be supported.
7.5 Technologies
Long-term goals are to improve efficiency, decrease environmental impact, and enhance the economic viability of advanced biofuel processes.
Technologies need to be developed in the context of “lifeline” needs and address the integrated system – from biomass production and conversion to end use of all products – for the specific sites where these technologies will be applied. These dramatically differ depending on the intended application and end use36. Knowledge on requirements for small and large-scale bioenergy production from bioprocess design should be combined with knowledge on innovation and financial management37.
There is need for investment in advanced biosciences research-genomics, molecular biology, and genetics – for major platforms – sugar, syngas, methane and other bioproducts for fuels, including hydrogen, and chemicals38. Careful consideration is needed to define how best biomass is used, converted, scaled up and deployed to an appropriate level and in understanding the potential value of every single stream of organic matter – a no waste philosophy39. The complete use of feedstocks must be sought to convert all primary energy content of the material to useful products40.
Continued innovation in use of waste materials and in water and nutrient reuse and recycling in bioenergy systems is needed to fulfill the potential contribution of biomass to sustainable energy production41.
Most processing technologies for first generation 1G biofuels are fully mature, but there is room for improvement, especially in the energy balance42. Higher efficiency technologies are needed for traditional bioenergy applications and continued development of integrated, resource-efficient biomass conversion pathways43. For instance, multi-fuel processing (e.g., co-combustion or co-firing of several biomass types in a single furnace) in flexible plants should be investigated based on the physicochemical properties of biomass collected, handled and sent to processing plants44.
Lignocellulosics are going commercial. Although cellulosic ethanol is undergoing initial scale commercial deployment, more work is needed to bring down the cost of the new conversion methods45. Improvements in microorganisms and enzymes are needed, especially for pentose fermentation, and in added value co-products (such as the use of lignin for energy and its fractions as source of value added co-products)46.
Advanced biofuels still require many technical and financial advances. Biofuels that would be suitable for jet fuel applications are highly desirable47 and in need of pilot and demonstration scale plants. Catalysts are needed with increased robustness and longevity and cost reduction in clean up. Flexible biorefinery systems need designing and engineering efforts48.
To be able to use the “new” opportunities of feedstock supply (such as urban residues, agricultural residues)49 there is need of advances in metagenomics. Vegetable oil production needs feedstock flexible processes50.
7.6 Exploring Social and Environmental Benefits
Among the renewable energy options, bioenergy is the system with more wide spread benefits. To better explore and enhance benefits associated with agriculture and rural development, such as improved energy and food security as well as opportunities to develop a whole new industry based on biomass, it is essential to develop monitoring and evaluation systems51, or use existing ones, to evaluate the progress towards sustainability on social and environmental issues52. There is need to improve datasets regarding good cases where projects clearly demonstrate the link between the reduction of energy poverty and greater energy access, through the improvement of livelihoods, and based on more effective and efficient use of biomass for energy. Because bioenergy impacts tend to be site-specific, not only more case studies are needed but also integrative approaches that can draw lessons from those case studies and from improved indicators and methodologies for tracking and monitoring progress53.
Data collection should be carried out according to carefully selected indicators used to measure the evolution of different aspects of environmental or socio-economic contexts. This will entail a larger effort that needs to involve producers, local governments and international organizations54. We need improved methodologies for estimating, quantifying, and verifying land use changes (LUC)55. Long-term research is needed on soil nutrient and carbon cycles under perennial crop and forest systems, and on how land use changes affect water and soils56. Improved methods should be developed to leverage remote sensing capabilities for monitoring land use and soil and water status57.
Understanding the dynamics of land use change, both direct and indirect, is very important for the assessment of several key impacts of biofuels. There is no consensus on the methodology to be used and there is a critical shortage of reliable, reasonably disaggregated data in time and space58. Research should aim to develop methods and generate data for a higher level of scientific consensus on the indirect land use change (iLUC) evaluation, and on the treatment of net emissions for co-products, by-products and N2O emissions data in bioenergy systems59. Further development of greenhouse gas life-cycle assessment (GHG LCA) methodologies is needed to quantify the influence of timing of emissions and removals, albedo change, and short-lived climate forcing agents. New data will be required in order to apply these methods60.
The next step in standards development must be the critical pursuit of the technical, scientific, and educational capacity to assess baseline conditions and tailor implementation solutions that provide measureable outcomes both at the sub-field, field, and landscape levels. These capacity needs are particularly pressing in the area of biodiversity, water quality, carbon accounting, and socio-economic conditions within broader communities. At least from a certification perspective, it is questionable whether additional research dollars should be spent in the pursuit of more indirect effects modeling. Instead, investments should be made in building capacities in public governance parallel to those for certification61.
1 (Chapter 3)
2 (Chapter 5)
3 (Chapter 12)
4 (Chapter 18)
5 (Chapter 13)
6 (Chapter 4, Chapter 5)
7 (Chapter 4)
8 (Chapter 20)
9 (Chapter 4)
10 (Chapter 20)
11 (Chapter 3)
12 (Chapter 10)
13 (Chapter 4)
14 (Chapter 9)
15 (Chapter 5)
16 (Chapter 10)
17 (Chapter 11)
18 (Chapter 4)
19 (Chapter 4)
20 (Chapter 18)
21 (Chapter 4)
22 (Chapter 6)
23 (Chapter 4)
24 (Chapter 13)
25 (Chapter 14)
26 (Chapter 5, Chapter 18)
27 (Chapter 10)
28 (Chapter 5)
29 (Chapter 10)
30 (Chapter 11)
31 (Chapter 10)
32 (Chapter 11)
33 (Chapter 11)
34 (Chapter 3)
35 (Chapter 2 section 2.2.4)
36 (Chapter 3)
37 (Chapter 4)
38 (Chapter 12)
39 (Chapter 12)
40 (Chapter 14)
41 (Chapter 18)
42 (Chapter 14)
43 (Chapter 3)
44 (Chapter 11)
45 (Chapter 3)
46 (Chapter 12)
47 (Chapter 3)
48 (Chapter 12)
49 (Chapter 6)
50 (Chapter 12)
51 (Chapter 16)
52 (Chapter 15)
53 (Chapter 16, Chapter 21)
54 (Chapter 15)
55 (Chapter 5)
56 (Chapter 18)
57 (Chapter 18)
58 (Chapter 14)
59 (Chapter 17)
60 (Chapter 17)
61 (Chapter 16, Chapter 19)
To cite this book
Souza, G. M., Victoria, R., Joly, C., & Verdade, L. (Eds.). (2015). Bioenergy & Sustainability: Bridging the gaps (Vol. 72, p. 779). Paris: SCOPE. ISBN 978-2-9545557-0-6